Integrated communications and sensing at mmWave and sub-THz frequencies could add location sensing to help avoid accidents between pedestrians, cyclists, and vehicles.
The commercial rollout of 5G is well underway and its capabilities are now available to users. Still, the telecommunications industry is laying the foundations for the next generation of cellular communications. 6G uses many technologies to bridge the digital and physical worlds through ubiquitous connectivity. Integrated Communications and Sensing (ICAS) – also known as Joint Communications and Sensing (JCAS) – is expected to be a core part of 6G. In-network sensing aims to leverage the large amount of network infrastructure already in place, making sensing available as a “free” utility that can improve connectivity between devices and between devices and humans.
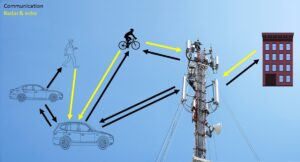
Figure 1. This conceptual diagram allows communications and sensing signals to pass between base stations, vehicles, and pedestrians.
Combining communication and sensing brings significant benefits to networks.Consider the diagram of Figure 1 and autonomous driving use cases. Radar signals can detect the precise location of objects around the car and share this information with other self-driving cars and networks. The combination of sensing and communication enables accurate positioning and spatial monitoring over 6G networks.
The idea of ICAS is simple. Detect data about the physical world by adding sensing signals to traditional communication signals. In theory, a communication signal can occupy a portion of the total allocated bandwidth. Sensing signals such as radar chirps and sounding signals can occupy the remainder of the allocated bandwidth. Combining these two signals into one waveform allows different applications to share antenna and transceiver hardware while occupying the same spectrum slice. Both spectrum and physical space within user equipment are finite resources, which can lead to creative techniques to maximize both. Research continues into the optimal waveform for sensing signals and the ideal ratio of sensing bandwidth to communication bandwidth.
Design considerations and challenges
For signals below 6 GHz, reducing the bandwidth of the communication signal is impractical. Maximizing data throughput remains a priority for these frequencies as they reach large areas covering both outdoors and indoors. In higher bands, the total available bandwidth in the gigahertz range works by giving a portion of that bandwidth to the sensing signal. Regardless of the frequency, there is always a trade-off between the resolution of the sensing signal and the throughput of the communication signal. For example, if a radar chirp is the sensing signal, to obtain a range accuracy of 15 cm he needs a bandwidth of 1 GHz. Some applications may require this level of accuracy or higher. Therefore, a “one size fits all” solution to the sensing and communication bandwidth challenges is unlikely. Flexible frame structures and reconfigurable numerology could potentially address that.
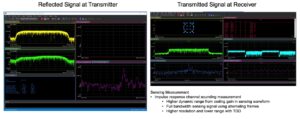
Figure 2. With TDD, a transmitter sends sensing and communication signals at different times, each capturing the entire bandwidth.
Consider the following two different waveform configuration examples.in Figure 2, the full bandwidth of the signal is used for sensing using alternating frames. This time-domain duplex (TDD) approach provides high sensing resolution. Unfortunately, the scope is limited.
In the second configuration (Figure 3), the narrow-bandwidth sensing signal is placed on the unused subcarriers of the OFDM signal. This frequency-division duplexing (FDD) approach allows for longer detection ranges, but with lower resolution. Neither approach is wrong, but this example illustrates the kind of trade-offs an engineer must consider when designing a transmitter and receiver for his ISAC waveform.
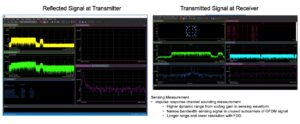
Figure 3. With FDD, sensing and communication signals are displayed simultaneously, but each occupies less bandwidth than TDD.
In addition to available bandwidth, higher frequencies also offer sensing advantages. For high-resolution radar, large K-band (18 GHz to 26.5 GHz) and Ka-band (26.5 GHz to 40 GHz) antennas have been installed for many years. This frequency overlap and the increasing number of large antenna arrays in communication systems opens up the possibility of reusing existing infrastructure and equipment for ICAS applications. Looking higher up the spectrum, automotive radars operating at 77 GHz have expanded in recent years, providing new opportunities to leverage and reuse existing infrastructure by adding sensing to wireless networks. .
Testing and characterization
Engineers need testing to better understand sensing performance at different frequencies. For example, you should compare and contrast sensing at different bandwidths He 24 GHz and He 77 GHz. This type of information will aid in the commercialization of ICAS technology. More fundamentally, we need a deeper understanding of the transmission channels from 7 GHz to 24 GHz to understand how sensing signals in combination with communication signals in the so-called FR3 band work. Existing channel models standardized by 3GPP lack details for this use case. NextG Alliance is currently collecting sounding data and studying different frequency bands to better understand channels in the context of sensing applications.
Traditional communication channel models do not account for reflections, so a model is needed to characterize the reflectance of sensed objects. We also need a model that takes into account different signal heights. This represents a complex physical world in which objects to be sensed exist, from people on the ground to unmanned aerial vehicles (UAVs) in flight. Finally, the industry needs a discussion about the use of dynamic and probabilistic models that account for target mobility and changing location in ICAS use cases.
conclusion
If the goal is to introduce sensing into the communications infrastructure, the communications industry must consider spectrum allocation and the amount of network resources required dedicated to sensing. There is a need to minimize resources from the network and maximize sensing. In addition, new waveform designs should be considered as waveform designs facilitate sensing. Finally, you should test at several frequencies to find the best frequency for this operation. Traditionally, sensing measurements have been made at high millimeter wave or terahertz frequencies. The feasibility of sensing within these bands needs to be established. Although technical questions abound, adding sensing to 6G networks is a promising feature that will make future networks more intelligent and enable many new applications.
For more information
Video: Toward unifying sensing and communications in 6G, Nokia, https://www.youtube.com/watch?v=BCKQHQY7hMI.
Video: VTC2023-Spring Keynote: Integrated Sensing and Communications: It was Meant to Be, University College London, https://www.youtube.com/watch?v=Y8oCRAqtUCk
Integrated Sensing and Communications: Enabling Technologies, Applications, Tools and Datasets, Standardization, and Future Directions, National Institutes of Health, https://www.ncbi.nlm.nih.gov/pmc/articles/PMC10236932/
Joint Communication and Sensing in 6G Networks, Ericsson, https://www .ericsson.com/en/blog/2021/10/joint-sensing-and-communication-6g
Sarah LaSelva leads Keysight’s marketing efforts in 6G. She has over 10 years of experience in test and measurement with an emphasis on wireless communications, both researching and disseminating the latest wireless technologies. Throughout her career, she has spent time in marketing, test engineering, and application engineering.
Sarah’s background is in microwave and millimeter wave technology. She holds a bachelor’s degree in electrical engineering from Texas Tech University.